The advent of 5G technology marks a significant milestone in the evolution of wireless communication. Among the most promising yet challenging aspects of this new generation is millimeter-wave (mmWave) technology, heralded for its potential to deliver unprecedented bandwidth and ultra-fast speeds. However, several years into the 5G rollout, mmWave continues to grapple with complex technical and economic hurdles. This comprehensive exploration aims to demystify the dark arts of Radio Frequency Integrated Circuits (RFICs) and shed light on the intricacies of mmWave deployment in the 5G landscape.
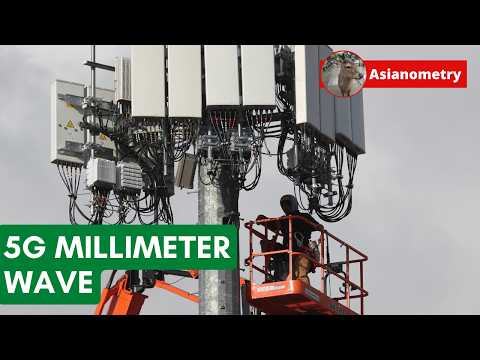
The Fundamentals of Wireless Communication
Understanding Waves, Frequency, and Wavelength
At the heart of wireless communication lies the concept of electromagnetic waves traveling through space. The wavelength of a wave is the distance it covers in a single cycle, measured in meters. Frequency, measured in hertz (Hz), denotes how many cycles pass a given point per second. The relationship between frequency and wavelength is inversely proportional: as frequency increases, wavelength decreases.
- Low Frequency: Longer wavelengths (e.g., radio waves with frequencies in kilohertz or megahertz).
- High Frequency: Shorter wavelengths (e.g., gamma rays with frequencies in the terahertz range).
The Electromagnetic Spectrum and Its Allocation
The electromagnetic spectrum encompasses a broad range of frequencies, each with specific applications:
- Low Frequencies: Used for long-distance radio communications.
- Mid Frequencies: Allocated for television broadcasts, GPS, and cellular networks.
- High Frequencies: Employed in satellite communications and medical applications like cancer treatment.
National regulatory bodies allocate portions of the spectrum for various uses, balancing the limited resource among competing needs such as radar, wireless communication, and Wi-Fi. The finite nature of the spectrum presents ongoing challenges in accommodating the ever-growing demand for wireless data transmission.
The Limitations of 1G to 4G Networks
Spectrum Constraints and Capacity Challenges
Previous generations of cellular networks (1G through 4G) predominantly operated below 7.125 gigahertz (GHz). Over time, these frequency bands became increasingly congested. Typically, a cellular operator might have access to only about 200 megahertz (MHz) of spectrum in a given area—insufficient for meeting the escalating data demands of modern users.
The Struggle with Bandwidth
Bandwidth—the range of frequencies available for transmitting data—is crucial for network capacity and speed. Limited bandwidth in the traditional frequency bands hindered the ability of 4G networks to deliver higher data rates and support a growing number of devices.
The Evolution to 5G: Redefining Wireless Communication
Philosophical Foundations of 5G
Engineers and policymakers envisioned 5G not merely as an incremental upgrade but as a transformative platform to address diverse communication needs. The 5G standard introduces flexibility in frequency band usage and aims to support a wide array of applications through three primary service categories:
- Enhanced Mobile Broadband (eMBB): Focused on delivering higher data speeds for activities like streaming high-definition video and virtual reality.
- Ultra-Reliable Low-Latency Communications (URLLC): Designed for mission-critical applications requiring minimal delay and high reliability, such as autonomous vehicles and remote surgery.
- Massive Machine Type Communications (mMTC): Tailored for the Internet of Things (IoT), connecting a vast number of low-power, low-data-rate devices like sensors and smart appliances.
Expanding into New Frequency Bands
To meet these ambitious goals, 5G expands into new frequency territories, including the mmWave bands ranging from 24.25 GHz to 52.6 GHz. This expansion opens up substantial new bandwidth but also introduces technical complexities.
Exploring Millimeter-Wave Technology
What Is Millimeter-Wave?
Millimeter-wave refers to the portion of the electromagnetic spectrum with wavelengths between 1 millimeter and 10 millimeters, corresponding to frequencies from approximately 30 GHz to 300 GHz. These high frequencies offer:
- Large Bandwidth: Enabling higher data transmission rates.
- Shorter Wavelengths: Allowing for smaller antennas and more compact equipment.
Historical Context: The Pioneering Work of Pi and Khan
In 2011, researchers Zhouyue Pi and Farooq Khan from Samsung Electronics published a seminal paper proposing the use of mmWave bands for future mobile communications. They argued that to overcome the limitations of existing networks, it was essential to exploit the untapped potential of higher frequency bands. Their work laid the groundwork for incorporating mmWave into the 5G standard.
Early Applications and Trials
Prior to 5G, mmWave frequencies were primarily used for:
- Military and Satellite Communications: Secure and high-capacity links.
- Automotive Radar: Enhancing vehicle safety systems at 77 GHz.
- WiGig Wi-Fi Standard: An attempt to utilize the 60 GHz band for high-speed wireless networking, which saw limited adoption.
Research in the early 2010s by institutions like NYU and UT Austin demonstrated the feasibility of using mmWave for consumer wireless applications, paving the way for its inclusion in 5G.
Challenges of Millimeter-Wave Technology
Technical Obstacles
Path Loss and Attenuation
One of the primary challenges with mmWave frequencies is path loss, the reduction in signal strength as it propagates through space. Path loss increases with the square of the frequency, meaning higher frequencies like mmWave experience significantly more loss than lower frequencies.
- Equation: The Friis Transmission Equation quantifies path loss, showing an inverse relationship with wavelength.
- Implication: mmWave signals require more power or advanced techniques to maintain signal strength over distance.
Line-of-Sight Requirements
mmWave signals struggle to penetrate obstacles such as buildings, foliage, and even human bodies. This limitation necessitates a line-of-sight (LOS) path between the transmitter and receiver, complicating network design in urban environments.
Environmental Factors
- Obstruction by Objects: Walls, glass, and other materials can significantly attenuate mmWave signals.
- Weather Conditions: While heavy rainfall has less impact than expected, other factors like humidity can affect signal propagation.
Economic and Infrastructure Challenges
Network Densification
To overcome the limited range and penetration issues, mmWave deployment requires a dense network of small cells:
- Small Cells: Low-power base stations covering areas as small as 50 to 100 meters.
- Deployment Complexity: Installing numerous small cells is time-consuming, costly, and often faces regulatory hurdles.
Site Acquisition and Aesthetics
- Power and Connectivity: Each small cell needs a reliable power source and a high-speed backhaul connection.
- Permitting: Obtaining the necessary permissions can be a bureaucratic challenge.
- Community Acceptance: Aesthetic concerns and health fears can lead to public resistance.
Radio Frequency Integrated Circuits (RFICs): The Heart of mmWave Communication
What Are RFICs?
Radio Frequency Integrated Circuits (RFICs) are specialized semiconductor devices that handle the transmission and reception of radio signals. They integrate various components like mixers, amplifiers, and oscillators into a compact chip.
The Complexities of RFIC Design
The Transceiver Process
- Data Conversion: Digital data from the device is converted into an analog baseband signal.
- Upconversion: The baseband signal is mixed with a higher-frequency carrier signal to create a passband signal suitable for transmission.
- Power Amplification: The passband signal is amplified to overcome path loss and ensure it reaches the receiver.
- Transmission: The amplified signal is sent through the antenna array.
Technical Considerations
- Linearity: Ensuring the amplifier maintains signal integrity without distortion.
- Efficiency: Balancing power consumption with performance to preserve battery life and manage heat dissipation.
- Integration: Combining multiple antennas and components in a compact form factor.
Antenna Design and Beamforming
Antenna Arrays
mmWave technology allows for smaller antennas due to shorter wavelengths. Devices can incorporate arrays of antennas (e.g., 32 or more) to improve signal strength and directionality.
Beamforming Techniques
- Directional Beams: Antenna arrays can focus the signal into narrow beams aimed at the receiver, enhancing signal quality.
- Adaptive Steering: The system can adjust the beam’s direction in real-time to maintain a connection as devices move.
Overcoming Technical Challenges
Mitigating Path Loss
Increased Power and Amplification
While simply boosting power is not always practical due to efficiency and regulatory limits, careful design of power amplifiers can improve performance.
Advanced Materials and Components
- Semiconductor Innovations: Utilizing materials like gallium nitride (GaN) can enhance amplifier efficiency and power handling.
- Low-Noise Amplifiers: Improving receiver sensitivity to detect weaker signals.
Enhancing Signal Propagation
Reflectors and Repeaters
- Strategic Placement: Installing passive reflectors on buildings to redirect signals around obstacles.
- Active Repeaters: Devices that receive, amplify, and retransmit signals to extend coverage.
Network Planning and Optimization
- Dense Small Cell Deployment: Increasing the number of base stations to reduce the distance between transmitters and receivers.
- Intelligent Algorithms: Using software to manage network resources and optimize connections.
The Deployment of mmWave 5G Networks
Infrastructure Requirements
Deploying mmWave networks involves significant changes compared to traditional cellular networks:
- Small Cell Sites: Thousands of new locations need to be secured, each requiring power and backhaul connectivity.
- Equipment Installation: Specialized hardware must be installed, often in challenging environments.
- Regulatory Compliance: Navigating local regulations and obtaining necessary permits.
Economic Considerations
- High Capital Expenditure: The cost of equipment, installation, and site acquisition is substantial.
- Return on Investment: Uncertainty about consumer demand and revenue potential makes the financial case challenging.
- Competitive Pressure: Operators must balance the need to innovate with the risk of overextending financially.
Real-World Deployments and Case Studies
The United States Experience
Verizon and AT&T’s Early Adoption
- Strategic Choice: Focused on mmWave due to existing low- and mid-band spectrum being occupied by legacy networks.
- Initial Rollout: Began deploying Non-Standalone 5G networks in 2018, targeting both mobile services and Fixed Wireless Access (FWA).
Challenges Faced
- Limited Coverage: mmWave’s short range led to spotty coverage, often confined to specific urban areas.
- Consumer Reception: Users were not always willing to pay premiums for higher speeds, especially with inconsistent availability.
- Device Support: The iPhone 12’s mmWave capability was limited to the U.S. market, hindering widespread adoption.
Shift in Strategy
- Acquisition of Mid-Band Spectrum: In 2021, both Verizon and AT&T invested heavily in acquiring C-band spectrum around 3.7 GHz.
- Focus on Mid-Band Deployment: Mid-band offers a balance of coverage and capacity, leading to a pivot away from mmWave emphasis.
Global Perspective
Limited Adoption Worldwide
- Japan’s Deployment: One notable exception, Japan invested in mmWave infrastructure ahead of the 2020 Tokyo Olympics.
- European Hesitance: European operators have been more cautious, focusing on mid-band deployments.
Factors Influencing Global Adoption
- Regulatory Environment: Spectrum allocation and licensing vary by country.
- Market Demand: Different consumer behaviors and expectations impact the perceived value of mmWave services.
- Infrastructure Costs: Economic considerations may deter operators in markets with lower revenue potential.
The Future of mmWave and 5G
Potential Applications
Despite current challenges, mmWave technology holds promise for specific use cases:
- High-Density Environments: Airports, stadiums, and concert venues where large numbers of users require high data rates.
- Fixed Wireless Access: Providing broadband connectivity in rural or underserved areas without the need for physical cables.
- Industry 4.0: Enabling advanced manufacturing processes with real-time data exchange and automation.
Ongoing Research and Development
- Technological Advancements: Companies like Qualcomm continue to improve mmWave capabilities, including better RFICs and antenna designs.
- Network Evolution: Transitioning to Standalone 5G networks may unlock more of mmWave’s potential through optimized infrastructure.
Challenges Ahead
- Finding the Killer App: A transformative application that drives mass adoption of mmWave technology has yet to emerge.
- Economic Viability: Operators must reconcile the high costs of deployment with uncertain returns.
- Consumer Perception: Overcoming skepticism and demonstrating tangible benefits to end-users.
Conclusion
The journey of mmWave technology in the context of 5G is a complex interplay of cutting-edge engineering, economic realities, and market dynamics. While the potential for ultra-fast speeds and massive bandwidth is undeniable, significant technical and logistical challenges remain. The success of mmWave will likely depend on targeted deployments where its advantages can be fully realized, continued technological innovation, and the emergence of compelling applications that resonate with consumers and industries alike.
As we stand at the cusp of this wireless frontier, the question remains: Will mmWave become a cornerstone of our connected future, or will it remain a specialized tool in the broader 5G toolkit? The answer may well shape the next decade of technological progress.
Exploring the Potential Health Effects of 5G and Wireless Radiation: A Comprehensive Review
The rollout of 5G technology has sparked a global conversation about the potential health effects of increased exposure to radiofrequency electromagnetic fields (RF-EMFs). While 5G promises faster speeds and more reliable connections, concerns have been raised about its impact on human health. Recent studies, including those from Tomsk State University and Yale University, have highlighted possible links between RF-EMF exposure and neurological changes in animals. This article delves into the latest research, connects it with existing studies, and examines what this means for public health and future technological developments.
Understanding the Concerns Surrounding 5G Radiation
The Rise of 5G and Public Anxiety
As 5G networks expand globally, they operate at higher frequencies than previous generations, leading to questions about the biological effects of this increased exposure. Public concern ranges from potential cancer risks to neurological impacts, especially on vulnerable populations like children and pregnant women.
The Difference Between 5G and Previous Generations
While earlier generations like 1G operated around 900 MHz frequencies, which can penetrate deeper into the body, 5G utilizes higher frequencies that are absorbed more superficially. Some experts argue that this could make 5G less harmful than its predecessors, but the scientific community remains divided.
Recent Studies Highlighting Potential Health Effects
Russian Study: 5G Radiation and Brain Changes in Rats
Key Findings:
- Objective: Investigate the effects of non-ionizing 5G radiation on rats of different ages.
- Methodology: Male Wistar rats were exposed to 5G RF-EMF frequencies for five weeks, equivalent to approximately four human years.
- Age Groups: Corresponded to human adolescents, adults aged 40 and up, and seniors aged 65 and older.
- Results: No outward behavioral changes were observed, but significant changes were found in the ratio of antioxidants and oxidants in the brain tissue of exposed rats.
Implications:
- The alterations suggest oxidative stress in the brain, potentially leading to cellular damage.
- Oxidative stress is associated with negative health outcomes, including neurodegenerative diseases.
- The study emphasizes the need for long-term research to determine cognitive effects and compensatory mechanisms.
Connecting to U.S. Research: Yale University’s Study on ADHD
Overview:
- Objective: Examine the effects of prenatal cell phone radiation exposure on the neurodevelopment of mice.
- Methodology: Pregnant mice were exposed to radiation from an active cell phone positioned above their cage throughout gestation.
- Results: Offspring exhibited behavioral hyperactivity and decreased memory capacity, attributed to altered development of neurons in the prefrontal cortex.
Implications:
- Suggests a potential link between prenatal exposure to cell phone radiation and ADHD-like symptoms.
- Highlights concerns about the safety of wireless device usage during pregnancy.
- Indicates that EMF exposure may interfere with normal brain development.
Dr. Martin Pall’s Research on Autism and EMF Exposure
Key Concepts:
- Voltage-Gated Calcium Channels (VGCCs): Pall suggests EMFs activate VGCCs, leading to increased intracellular calcium levels.
- Oxidative and Nitrosative Stress: Elevated calcium can produce excessive nitric oxide and superoxide, forming peroxynitrite, a potent oxidant.
- Neurodevelopmental Impact: This stress may disrupt normal neurodevelopmental processes, potentially contributing to autism spectrum disorder (ASD).
Supporting Evidence:
- Correlation with EMF Exposure: Epidemiological data shows increased autism prevalence in areas with higher EMF exposure.
- Animal Studies: Indicate that EMF exposure can alter brain development and function in animal models.
Calls to Action:
- Dr. Pall advocates for stricter regulatory standards and public health policies to reduce EMF exposure, especially among pregnant women and children.
Synthesizing the Findings: A Convergence of Concerns
Common Threads in the Research
- Oxidative Stress: Both the Russian study and Dr. Pall’s research highlight oxidative stress as a key factor in EMF-induced biological effects.
- Neurodevelopmental Disruption: The Yale study and Dr. Pall’s work suggest that EMF exposure can interfere with normal brain development, leading to behavioral and cognitive disorders.
- Vulnerability of the Young: All studies emphasize that younger organisms are more susceptible to potential adverse effects of EMFs.
Implications for Public Health
- Precautionary Measures: Given the potential risks, adopting precautionary principles in the use of wireless technology is prudent.
- Regulatory Reassessment: Current safety guidelines may need re-evaluation in light of emerging evidence.
- Further Research: There’s a critical need for comprehensive, long-term studies on chronic EMF exposure in humans.
The Challenge of Interpreting Scientific Evidence
Limitations and Considerations
- Animal Studies vs. Human Health: While animal studies provide valuable insights, results may not directly translate to humans.
- Conflicting Research: Some studies show no significant health effects from EMF exposure, highlighting the need for more robust research.
- Exposure Levels: Many studies use exposure levels higher than what most people experience, which may not reflect real-world scenarios.
The Role of Regulatory Bodies
- World Health Organization (WHO): Classifies RF-EMFs as “possibly carcinogenic to humans” (Group 2B), indicating limited evidence.
- International Commission on Non-Ionizing Radiation Protection (ICNIRP): Provides guidelines on exposure limits, but critics argue they may be outdated.
- Calls for Updated Standards: Advocates urge regulatory bodies to consider non-thermal effects and long-term exposure in their guidelines.
Balancing Technological Advancement and Health
The Necessity of Wireless Communication
- Economic Growth: 5G technology is pivotal for the development of smart cities, IoT devices, and improved connectivity.
- Societal Benefits: Enhanced communication networks support healthcare, education, and emergency services.
Mitigating Potential Risks
- Implementing Safety Measures:
- Use wired connections when possible.
- Keep devices away from the body, especially for children.
- Limit exposure time to wireless devices.
- Educating the Public: Increase awareness about safe technology use and potential risks.
- Encouraging Responsible Deployment: Advocate for careful placement of 5G infrastructure away from schools and hospitals.
The Future of Wireless Technology and Health Research
Emerging Technologies: From 5G to 7G
- Higher Frequencies: Future networks may utilize terahertz (THz) frequencies, potentially offering faster data transmission with even less penetration depth.
- Li-Fi Technology: Uses light waves for data transmission, which could reduce RF-EMF exposure indoors.
The Need for Ongoing Research
- Longitudinal Studies: Track health outcomes over extended periods to assess chronic exposure effects.
- Interdisciplinary Collaboration: Combine expertise from biology, engineering, and public health to understand EMF impacts fully.
- International Cooperation: Share data and resources globally to build a comprehensive understanding.
Conclusion
The potential health effects of 5G and wireless radiation remain a complex and evolving area of study. Recent research highlights possible links between RF-EMF exposure and changes in brain tissue, oxidative stress, and neurodevelopmental disorders. While definitive conclusions cannot yet be drawn, the convergence of evidence underscores the need for caution, further research, and updated regulatory standards.
As society becomes increasingly reliant on wireless technology, balancing the benefits of connectivity with the responsibility to protect public health is crucial. By staying informed, advocating for rigorous scientific investigation, and adopting prudent usage practices, we can navigate the uncertainties and work towards a future where technological advancement and health coexist harmoniously.