Our brains are incredibly selective when it comes to storing memories. Some experiences remain vividly imprinted for years, while others slip away within days or weeks. Have you ever wondered why you can recall exactly where you were and what you felt when something extraordinary happened—like winning a lottery—but struggle to remember ordinary moments like what you wore last Tuesday?
In this post, we’ll explore the fascinating cognitive processes that determine what we remember and what we forget. We’ll take a deep dive into the hippocampus, the seahorse-shaped brain structure responsible for forming new memories, and we’ll learn how sharp-wave ripples act as the brain’s internal bookmarking system. Drawing on recent research published in Science, we’ll see how memories are tagged for importance during wakefulness and later consolidated during sleep. This process, it turns out, is not just relevant for rodents in a laboratory maze—it’s a universal principle that underscores how humans and animals alike learn from experience, encode essential information, and refine skills over time.
Whether you’re a neuroscience enthusiast, a student of psychology, or someone curious about how learning and memory work, this discussion promises new insights. Let’s embark on this journey to uncover how your brain chooses what to remember.
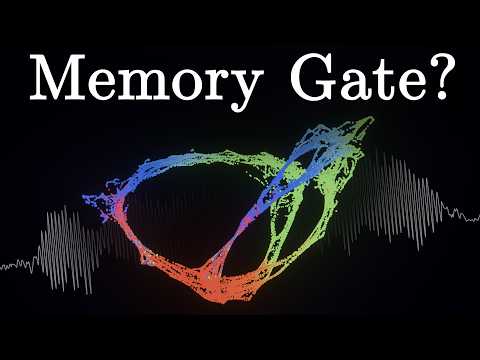
The Everyday vs. the Extraordinary
Think about the last time you had a routine breakfast. Chances are, you don’t remember every detail—what your coffee smelled like, how the toast tasted, or where you placed your spoon. However, if something truly extraordinary happened this morning—say, you received an unexpected job offer or found out you won a lottery—you’d likely remember a surprising level of detail for a very long time.
This reveals a fundamental phenomenon: the brain does not treat all experiences equally. It prioritizes those events that have significant emotional, survival, or novel value. Routine events, in contrast, fade away unless they are repeated or become relevant in some other context.
Why Some Memories Fade Quickly
- Limited Cognitive Resources: The brain’s storage capacity, while large, is not infinite. Maintaining every piece of information would be energetically wasteful.
- Use It or Lose It: Neural connections that are not repeatedly activated tend to weaken over time, a process often summarized by the phrase, “neurons that fire together, wire together.”
- Relevance and Emotion: Emotional salience or personal importance acts like a spotlight that helps the brain pick out which memories to reinforce.
Although researchers are still unraveling all the intricacies, it’s clear that selective memory is neither a flaw nor a bug in the system. Instead, it’s how our brains navigate an overload of information and extract the most vital pieces to carry forward.
Why Memory Selection Matters
Real-Life Consequences
Memory selection ensures we can:
- Focus on what is most relevant: By filtering out the mundane, the brain frees itself to concentrate on important lessons or threats.
- Adapt to new environments: Remembering how to solve a new puzzle or navigate a new city helps us learn and adapt quickly.
- Develop and refine skills: Repeated practice of an instrument or a sport gets stored more permanently due to the brain’s emphasis on relevant skill-building memories.
Connections to Mental Health
- Trauma and PTSD: In some cases, the brain prioritizes emotionally overwhelming memories so strongly that they become intrusive.
- Depression and Anxiety: Negative biases can cause certain types of memories to be overly selected for recall, influencing mood and mental health.
Hence, understanding why and how the brain picks certain moments to remember is a key challenge in neuroscience—and it has a wealth of practical applications.
Meet the Hippocampus: A Memory Powerhouse
Nestled deep within the temporal lobes of the brain, the hippocampus is crucial for what researchers call episodic memory—the ability to store and retrieve events that occur in a specific sequence and context. If you recall what you had for lunch yesterday, where you had it, and who you were with, you can thank your hippocampus.
Two Distinct Modes of Operation
- Encoding During Wakefulness
During your day, the hippocampus is in an “online” mode—observing, recording, and integrating new experiences into what is sometimes called a cognitive map. This map stores spatial information (like where the kitchen is located) and temporal order (the sequence of events in your day). - Replay During Sleep
When you fall asleep, the hippocampus shifts modes. Sensory input is significantly reduced, freeing the hippocampus to reactivate or “replay” experiences from your waking hours. This offline replay is believed to strengthen and reorganize these memories, gradually integrating them into more permanent storage locations in the neocortex.
The hippocampus, therefore, is not just a passive storage unit; it’s more like a dynamic hub that shapes how your brain records, replays, and prioritizes the events of your life.
Sharp-Wave Ripples: Your Brain’s Bookmark System
One of the most fascinating aspects of the hippocampus’ memory process is the phenomenon of sharp-wave ripples (SWRs). These are high-frequency bursts of synchronized neural activity, often described as resembling a “ripple” of electricity sweeping through the hippocampus.
What Are Sharp-Wave Ripples?
- Sharp Wave: A large, slow wave of electrical activity that sweeps through the hippocampus, often triggered by synchronized input from upstream areas.
- Ripple: A superimposed, high-frequency oscillation that occurs on top of the sharp wave. Inhibitory and excitatory neurons coordinate rapidly, causing a short, high-frequency burst of activity.
Together, sharp-wave ripples allow the brain to compress and replay recent experiences in a fraction of the original time. A sequence that took seconds or even minutes during the day might be replayed in just 100 milliseconds during a ripple event.
Competition and Selection
Not all memories get replayed equally. During a sharp-wave ripple, many neurons become activated, but inhibitory interneurons step in as gatekeepers, limiting which subsets of neurons can fire together. This sets up a competition in which the strongest (i.e., most important or most heavily reinforced) neural pathways “win out” and get replayed.
By the time the dust settles, only the memory traces that had been most robustly wired during the day—often because they were more salient or had a strong emotional or reward component—have been reactivated. This process ensures the memory is tagged for further strengthening during subsequent sleep-based consolidation.
Memory Consolidation: A Two-Stage Process
Stage 1: Awake Bookmarking
- Certain events—those that carry strong emotional significance, novelty, or reward—trigger awake ripples soon after they occur. These ripples appear to “mark” or “bookmark” these experiences, flagging them as high priority.
Stage 2: Sleep Replays
- When you sleep, the hippocampus goes into a more dedicated replay mode. The awakened patterns from Stage 1 are reactivated multiple times, leading to synaptic plasticity in the neocortex. Over repeated nights, these memories shift from a hippocampus-dependent state to a more distributed cortical network, making them more stable and long-lasting.
Interestingly, sleep provides the perfect environment for consolidation because the neocortex is in a receptive brain state, free from much of the noise and distractions of wakefulness.
Awake Ripples vs. Sleep Ripples
The Puzzle of Awake Replays
Previous research discovered that awake ripples exist, particularly during brief moments of rest or when an animal (or person) pauses a task. The question was: why generate a replay when the rest of the brain (especially the neocortex) is not yet in the ideal state to solidify that memory?
Recent findings show that awake ripples act like memory bookmarks. They occur immediately or shortly after significant events—reward moments, novel stimuli, or emotionally charged experiences—and mark those experiences for subsequent consolidation during sleep.
Why Not Consolidate Immediately?
- Neocortical Readiness: The neocortex needs to be in a more plastic, synchronized state that typically arises during deep (slow-wave) sleep to effectively incorporate new memories.
- Repeated Replays: Consolidation usually requires multiple replays to embed a memory into long-term storage networks.
- Processing New Information: During wakefulness, the hippocampus is busy encoding new experiences. It can’t consistently dedicate its resources to repeated replays without missing out on real-time encoding needs.
Thus, it makes sense for the brain to “tag” memories using awake ripples, then offload them into more permanent storage during subsequent sleep.
Experimental Setup: The Figure 8 Maze
One of the studies shedding light on these processes involved an elegant experiment with rodents navigating a Figure 8 maze:
- Task: Mice learned to alternate between the left and right arms of the maze to receive rewards. If a mouse found a reward on the left arm during one trial, it would need to head to the right arm on the next trial.
- Neural Recordings: Researchers recorded from hundreds of neurons in the hippocampus while mice performed this task repeatedly over several days.
- Sleep and Learning: Between each day’s trials, the mice slept. During this sleep, scientists observed how often sharp-wave ripples containing maze-related “replays” occurred.
Over time, the mice got better at the task, and their hippocampal recordings began to reflect these improvements through distinct neural firing patterns.
UMAP & Population Activity
When you have hundreds of neurons firing simultaneously, each with its own spike rate, it’s akin to capturing an entire orchestra playing together, where each neuron is like a separate instrument. Making sense of this rich “symphony” is complex.
Why Dimensionality Reduction?
In the maze experiment, each neuron’s firing rate could be considered one dimension of data. With hundreds of neurons, you end up with a high-dimensional dataset—often 400+ dimensions. Directly visualizing activity in 400 dimensions is impossible.
That’s where techniques like UMAP (Uniform Manifold Approximation and Projection) come in:
- Identify Similar Patterns: UMAP assesses which neural firing patterns are similar to one another in high-dimensional space.
- Construct a Graph: It creates a graph capturing these relationships.
- Preserve Local Structure: UMAP then maps this high-dimensional graph down to just a handful of dimensions—often 2D or 3D—while trying to preserve the “closeness” relationships between points as faithfully as possible.
With UMAP, researchers can visualize clusters or trajectories of neural activity that would otherwise remain hidden in a 400-dimensional “fog.”
Mapping the Maze Manifold
Applying UMAP to the hippocampal recordings while mice actively ran through the Figure 8 maze produced a remarkable looped structure that mirrors the maze layout:
- Spatial Location: If each point in the UMAP visualization is colored by the rodent’s actual position in the maze at that moment in time, the 2D or 3D plot reveals a shape that looks strikingly similar to the Figure 8 path.
- Temporal Progression: Color-coding points by trial number (or by how far along the mouse is in training) reveals how neural patterns change systematically as the animals learn.
This “maze manifold” effectively shows how the brain’s population activity organizes itself. The fact that UMAP discovered the shape of the maze just from neural activity (with no knowledge of the actual physical layout) confirms that hippocampal activity encodes both location and context of the task.
Decoding Ripple Content: What the Brain Replays
With this UMAP-derived manifold in hand, scientists can look at the hippocampal activity during sharp-wave ripples and see where (or when) those patterns map back onto the Figure 8 representation.
Awake Ripples at the Reward Site
- When the rodent pauses to consume a reward, it often experiences a short burst of awake ripples.
- By projecting the ripple-associated activity onto the manifold, researchers see that these bursts replay the specific trajectory the animal just ran—essentially re-running the path to the reward in compressed form.
Sleep Ripples and Post-Task Replays
- During post-task sleep, the same trajectories appear in the ripple data.
- These sleep ripples replay similar or identical sequences of activity, reinforcing the memory.
- Before the rodents have learned the task, sleep ripples do not show these patterns—indicating the replay is specific to newly acquired experiences that the hippocampus has deemed “important.”
In other words, we can decode exactly which part of the maze the brain is “thinking about” during these ripple events. The fact that awake replay predicts future sleep replay cements the idea that awake ripples serve as a memory tagging mechanism, ensuring these events are targeted for deeper consolidation later on.
Putting It All Together: The Memory Tagging Mechanism
- Encounter an Important Event: The mouse finds a reward in the left arm of the Figure 8 maze for the first time.
- Awake Ripple: Immediately after, the hippocampus generates a sharp-wave ripple. This ripple replays the neural sequence associated with the path to the reward, marking it as “significant.”
- Bookmark Created: The replay triggers local synaptic changes, effectively tagging that pathway so it’s more likely to be reactivated during sleep.
- Sleep: During slow-wave sleep, the hippocampus replays the same event (sometimes multiple times). The replay is now accompanied by synchronized neural states in the neocortex, which strengthen and store the memory in more distributed networks.
- Consolidation Complete: Over repeated nights, the memory transitions from a fragile state, heavily dependent on the hippocampus, to a more stable representation in the cortical circuits.
From the perspective of human memory, the same logic applies. When you experience a memorable event—like a major life milestone, a strong emotional moment, or a highly surprising outcome—your brain will likely generate awake ripples that mark the memory. Then, during sleep, repeated replay cements it.
Beyond the Maze: Wider Implications for Learning
Implications for Human Learning
Although much of this cutting-edge research is done with rodents, the principles of memory tagging and sleep-based consolidation extend to humans. Studies using functional MRI (fMRI) and electroencephalogram (EEG) in people have shown similar replay phenomena during naps or overnight sleep, often correlating with improved memory performance the next day.
Educational Applications
- Spaced Repetition: The act of reviewing material just before sleep and then revisiting it periodically exploits this two-stage consolidation process.
- Reward and Motivation: When a student feels rewarded—either through positive feedback or personal interest—the hippocampus might tag those lessons, increasing the likelihood of memory replay and retention.
- Sleep Hygiene: Chronic sleep deprivation can disrupt the very consolidation processes we rely on to store important information. Ensuring enough high-quality sleep is essential for robust learning.
Mental Health and Therapy
- Trauma Exposure: Therapies that address traumatic memories sometimes aim to alter how these memories are replayed, potentially reducing their emotional intensity.
- Sleep Interventions: Improving sleep quality can enhance learning, reduce anxiety, and aid in recovery from stress.
The broader picture is that the fundamental tagging and replay mechanisms in the hippocampus have ripple effects across an array of cognitive and emotional domains.
Conclusion: Key Takeaways and Final Thoughts
Summary of the Main Points
- Selective Memory: Our brains are wired to prioritize extraordinary or significant events over mundane ones.
- Hippocampal Roles: The hippocampus is central to episodic memory, operating in distinct modes—encoding during wakefulness and replaying during sleep.
- Sharp-Wave Ripples: These bursts of synchronized activity act like bookmarks, tagging important daytime memories for further consolidation.
- Awake vs. Sleep Ripples: Awake ripples occur immediately after meaningful events to earmark them, while sleep ripples re-express these memories in compressed form to strengthen neocortical storage.
- Experimental Insights: Studies in rodents running a Figure 8 maze have provided direct evidence of these replay events and revealed how they map to the actual tasks through dimensionality reduction tools like UMAP.
- Implications for Humans: The same processes underlie much of our learning, from everyday tasks to complex skill acquisitions, underscoring the importance of restful sleep and well-timed review of material.
Final Reflection
Understanding how your brain chooses what to remember underscores the remarkable efficiency of our minds. Rather than overwhelm us with every detail of daily life, our memory systems focus on events that matter. This not only saves mental energy but also ensures crucial experiences get robustly encoded for future use. However, the process is delicate and can be influenced by factors like emotional state, sleep quality, and the timing of rest intervals.
If you take away one core message from this exploration, let it be this: your brain has an elegant two-step system for memory. First, it tags events during the day, highlighting the ones that seem important. Then, during sleep, it revisits and solidifies these memories. Therefore, one of the best ways to boost your own memory—whether for work, school, or personal growth—is to pay attention to when you learn (ideally paired with rewarding, meaningful experiences) and how well you sleep afterward.
Call to Action
- Practice Mindful Study: Deliberately turn your attention to significant facts or concepts you want to remember, increasing the likelihood of hippocampal tagging.
- Prioritize Sleep: Aim for consistent, high-quality sleep to give your brain the uninterrupted replay cycles it needs for consolidation.
- Reflect on Rewards: After learning something or achieving a milestone, pause and give yourself a mental (or real) reward. This might encourage awake ripples that tag memories as important.
By harnessing these principles, you can tune into the natural rhythms of your brain’s memory system and optimize your learning in a profound and lasting way.
Additional Resources
- Born, J., & Wilhelm, I. (2012). “System consolidation of memory during sleep.” Psychological Research, 76(2), 192–203.
- Wilson, M. A., & McNaughton, B. L. (1994). “Reactivation of hippocampal ensemble memories during sleep.” Science, 265(5172), 676–679.
- Girardeau, G., & Zugaro, M. (2011). “Hippocampal ripples and memory consolidation.” Current Opinion in Neurobiology, 21(3), 452–459.
These studies provide additional background on how replay and consolidation were first discovered and how they continue to be an active area of research.
Final Note: Exploring Data on Your Own
As mentioned in the video, the authors of the recent Science paper have made some of their data available through an interactive website and a Colab notebook, allowing you to explore the plots and see exactly how hippocampal activity unfolds during tasks and during rest. If you’re interested in a hands-on look at the data—and possibly in applying advanced tools like UMAP to decode neural patterns—be sure to check out their supplementary materials and tutorials.
Remember: Science progresses by the curiosity and creativity of people willing to delve deeper. Whether your goal is academic research, personal enrichment, or simply understanding how your mind works, exploring these datasets can be a fantastic learning experience.
Thank you for reading, and here’s to sharper memories, better learning, and the ongoing marvel of how our brains manage to store the most important details of our lives. If you found this post insightful, consider sharing it with others who might be intrigued by the elegant dance of neuronal activity shaping what we remember—and what we forget.